Every living thing on Earth, from giant trees and whales to tiny bacteria, has one surprising thing in common: they all rely on carbon. It’s a bit of a mystery when you think about it. Carbon isn’t the most common element around; that title goes to oxygen. It’s not the most stable, like helium, and it’s far less abundant than other elements, like nitrogen which fills most of our atmosphere. But still, carbon is everywhere in us, making up a fifth of our bodies. So, why did life choose carbon, especially when there were other elements in greater supply? Let’s dive into the story behind why nature might have picked carbon as its building block of choice.
Out of the 94 naturally occurring elements on the periodic table, it’s intriguing to ponder why life predominantly hinges on carbon. With such an array of elements available, what makes carbon so special that life doesn’t gravitate towards something else?
The choice of carbon as the primary building block for life is rooted in its unique chemical and physical properties. Here are some key reasons why life is based on carbon rather than other elements:
Reasons why all life [as we know it] is based on carbon
1. Versatility in Bonding
Carbon stands uniquely poised in the periodic table, making it a primary candidate for forming the intricate molecular structures essential for life’s complex chemistry. But what grants carbon this exceptional ability? To delve into this, we must turn to the foundational principles of chemistry, rooted in physics, more specifically in quantum mechanics. Quantum mechanics dictates that certain electron orbital configurations are energetically more favorable, translating to greater stability. The epitome of this stability is showcased by the Noble gases, positioned on the far-right side of the periodic table. Their complete outer shell of electrons represents the most energetically efficient state, rendering them chemically inert.
Now, when we observe the periodic table, it’s systematically structured to indicate the maximum number of bonds an element can form by its column. Progressing from the left, the bond capacity increases from one to four bonds as we traverse the columns, and then decreases thereafter. Carbon, intriguingly, sits in the column where elements can form a maximum of four bonds. With six electrons in total, four of which reside in its outer shell, carbon aims to emulate the noble gas Neon by sharing or borrowing four more electrons. This four-sided bonding capacity can be likened to a Lego brick connecting from all four sides, enhancing building possibilities.
![Reasons Why Life is Based on Carbon Instead of Silicon: Carbon [C] sits in the column where elements can form a maximum of four bonds in the Periodic table of the elements.](https://cdn-0.ourplnt.com/wp-content/uploads/2023/09/periodic-table-of-elements-1024x597.webp)
Because of this, carbon can seamlessly form complex molecular configurations, from non-repetitive polymer chains to closed rings, to single, double, or even triple bonds with diverse elements. Such versatility leads to a myriad of possible molecular structures. This is evident in DNA, where carbon’s capability to handle intricate arrangements allows for the storage of vast biological information. In contrast, oxygen, albeit being Earth’s most abundant element, can only form two bonds, limiting its structural potential. Elements like boron, though potentially intriguing due to their three-bond capacity, are scarce, making carbon’s abundance and versatility indispensable for life’s complex demands.
2. Chain Formation and Stability of Carbon Compounds
The capacity for chain formation exhibited by carbon is foundational to its central role in the building blocks of life. Carbon atoms have a unique characteristic wherein they can bond with one another in a linear fashion, forming chains, or close upon themselves to generate rings. These chains and rings can range from the simplest of structures to intricate, sprawling webs of interconnected atoms.
But what truly sets carbon apart is its potential to form diverse combinations when creating these chains and rings. It can bond with itself in single, double, or even triple bonds, resulting in a plethora of molecular geometries and complexities. This vast array of configurations enables the formation of complex organic molecules such as carbohydrates, fats, proteins, and nucleic acids, which are pivotal for life as we know it.
Furthermore, these carbon-based chains and rings can integrate atoms of other elements, like oxygen, nitrogen, and sulfur, thereby producing even more molecular diversity. For instance, the carbon rings in glucose molecules are vital for energy storage, while carbon chains in fatty acids are crucial for cell membrane structures.
In contrast, other potential life-forming elements, like silicon, do not exhibit the same proficiency in creating varied and long chains or rings. Such a diverse molecular repertoire gives carbon a definitive edge, allowing it to support the manifold processes and systems seen in life. Without carbon’s unparalleled chain and ring formation abilities, the rich tapestry of biological complexity on Earth would be inconceivable.
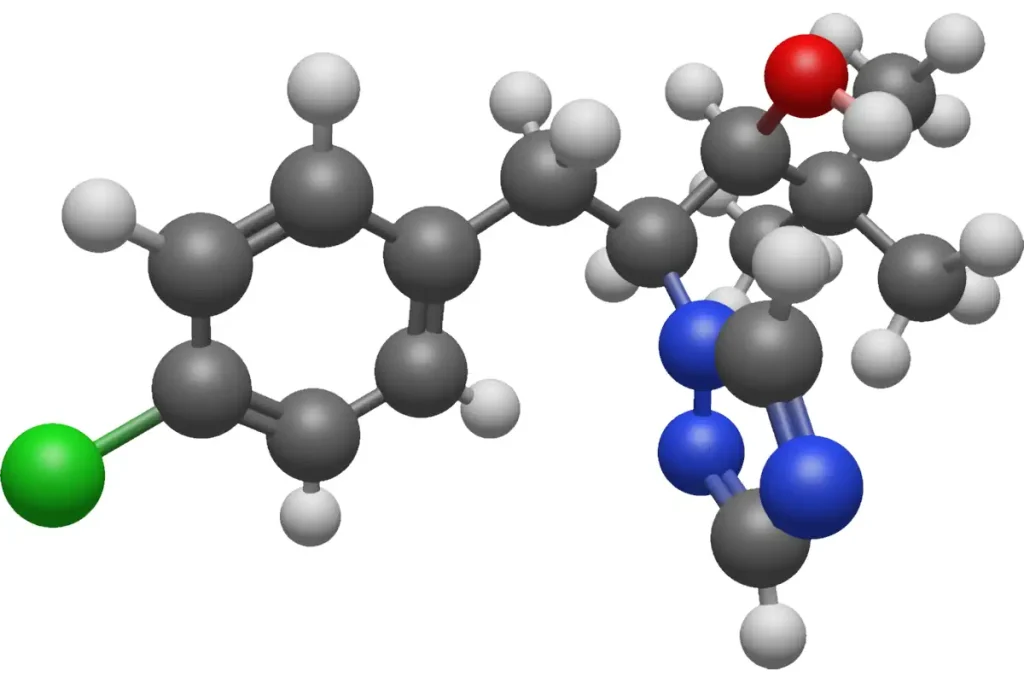
Why carbon can form long chain formations?
Carbon’s ability to form long chain formations and complex molecules stems from its electronic configuration and its unique position on the periodic table. Let’s break down what enables carbon to build such formations:
- Valence Electrons: Carbon has four valence electrons in its outer shell. This means it has precisely the right amount of room to form four covalent bonds with other atoms. This number of bonds is central to its versatility.
- Tetravalency: The four valence electrons enable carbon to be tetravalent, meaning it can form four bonds. These can be with other carbon atoms or different elements. This tetravalency allows carbon to create a diverse array of structures, from simple chains to complex branching trees or rings.
- Bond Strength: Carbon-carbon (C-C) bonds are strong yet flexible, making them suitable for forming stable chains or rings that can also be modified or broken under the right conditions (such as in metabolic reactions).
- Multiple Bonding Modes: Carbon is capable of forming single, double, and triple bonds. This versatility in bonding types allows for a vast range of molecular shapes and configurations. For example, the double bonds in certain carbon compounds introduce kinks or bend in the chain, leading to different molecular properties.
- Bond Angles: The angles at which carbon atoms bond in certain configurations (like the tetrahedral shape in methane or the planar shape in ethene) are conducive to forming long, stable chains or rings. These spatial arrangements allow molecules to minimize repulsive forces between electron clouds, leading to more stable structures.
When you couple these intrinsic properties of carbon with its ability to bond with a multitude of other elements (like hydrogen, oxygen, nitrogen, and sulfur), you get a recipe for an almost limitless array of molecules. These compounds can be linear, branched, or cyclic; saturated or unsaturated; simple or functionally diverse. This incredible adaptability of carbon to form a myriad of stable, complex structures is foundational to the existence and evolution of life as we know it.
Silicon is also tetravalent. But why can’t it form long chain compounds like carbon?
Silicon, despite being directly below carbon in the periodic table and sharing some similarities, does not readily form the long and complex chains as carbon does. Here’s why:
- Bond Strength: Silicon-silicon (Si-Si) bonds are weaker than carbon-carbon (C-C) bonds. This means that long chains of silicon atoms are inherently less stable and more prone to breaking than equivalent chains of carbon atoms.
- Size of the Atom: Silicon atoms are larger than carbon atoms. This larger size means that silicon atoms don’t pack as closely together as carbon atoms do, resulting in weaker bonds when silicon atoms form chains.
- Oxidation: Silicon readily reacts with oxygen to form silicon dioxide (SiO2). Unlike carbon, which can form a vast array of stable compounds with oxygen (like alcohols, aldehydes, acids, etc.), silicon’s primary oxide is a solid, non-reactive material (commonly seen as sand). This propensity to form SiO2 rather than complex chains limits silicon’s versatility in potential biochemistry.
- Multiple Bonding: Silicon has difficulty forming multiple bonds (like double and triple bonds) with itself or other atoms. While carbon can easily form double and triple bonds, which greatly increases the diversity and complexity of its compounds, silicon compounds are generally more limited in variety.
- Hybridization: One of the reasons carbon can form a diverse range of compounds is due to its ability to undergo various forms of hybridization (sp, sp2, sp3) which allows it to form different types of bonds and molecular structures. Silicon, due to its larger atomic size and electron distribution, doesn’t exhibit this property to the same extent.
While silicon-based life is a popular trope in science fiction, given our current understanding of chemistry, carbon offers versatility and stability that silicon cannot match, making carbon far more suitable as the foundational element for the complex chemistry of life.
3. Energy Efficiency
At its core, life is a series of chemical reactions where molecules are transformed, built up, and broken down. These reactions require energy, and likewise, they can produce energy. Carbon-based compounds, especially in the context of biological systems, are adept at both storing and releasing energy in controlled and efficient ways.
- Chemical Bonds and Energy: When we say that a molecule “stores” energy, we’re generally referring to the energy contained within its chemical bonds. Breaking certain chemical bonds releases energy while forming other bonds requires energy. In biological systems, the energy contained in the bonds of specific carbon-based molecules, like glucose, can be released in controlled environments, such as cellular respiration in our cells.
- ATP – The Energy Currency: The energy released from breaking down carbon compounds is often stored in the form of ATP (adenosine triphosphate). This molecule is like the energy currency of the cell. When carbon compounds are metabolized, the energy released is used to produce ATP, which the cell can then use to power other vital processes.
- Controlled Release: The beauty of carbon-based molecules, like sugars and fats, is that they don’t just combust or explode spontaneously. Instead, they require specific conditions and enzymes to break down in a regulated manner. This controlled release ensures that energy is made available to organisms when they need it, without wasting it all at once.
- Efficient Storage: Carbon compounds, especially lipids (fats), are incredibly dense energy storage molecules. This means that organisms can pack a lot of energy in a small space, making it efficient for both mobility and survival during times when food is scarce.
Metabolism encompasses all the chemical reactions in a living organism that produce or consume energy. This includes breaking down nutrients to extract energy (catabolism) and building up compounds for growth and repair (anabolism). For these processes to run smoothly, there must be a reliable and efficient source of energy. Carbon-based compounds, by virtue of their chemical structures and the bonds they contain, provide this source. They can be metabolized in cellular reactions to produce energy exactly when and where it’s needed, ensuring the organism’s survival and proper functioning.
In summary, the energy efficiency of carbon-based compounds plays a pivotal role in supporting the myriad of metabolic processes that underpin life. Their ability to store vast amounts of energy and release it in a controlled manner is a cornerstone of biochemistry and cellular function.
4. Carbon’s Abundance in the Universe
Carbon’s place in the universe is not by mere accident. When we survey the elemental composition of our solar system, it becomes evident that carbon ranks high. Among the top five most abundant elements, carbon takes the fourth spot, after hydrogen, helium, and oxygen, followed by nitrogen. This hierarchy provides an interesting reflection when we compare it to the composition of the human body. Here, carbon also emerges as one of the dominant elements, standing at the second position after oxygen. This parallel is telling. It suggests that there’s a natural alignment between the universe’s composition and the makeup of life, especially when we consider that four of the top five elements in our solar system are also fundamental to our body’s composition.
The Abundance Factor in Life’s Building Blocks
Abundance is crucial when considering the building blocks of life. An analogy would be the importance of having enough Lego bricks when trying to build a castle. In the same vein, the sheer availability of carbon in the universe makes it a logical choice as life’s foundational element. If carbon were a rarity, the odds of life as we know it emerging would be substantially lower. The fact that carbon is both versatile in its bonding abilities and abundant makes it a prime candidate for crafting the complex structures necessary for life.
Silicon, Nitrogen, and Abundance
One might wonder about other elements that show promise. Silicon, for instance, is abundant and can form four bonds similar to carbon. And yet, life is predominantly carbon-based, not silicon-based. Nitrogen, while forming only three bonds, is plentiful, constituting 78% of our atmosphere. But the abundance of an element alone isn’t the only criterion. The element also needs the right properties to support the complexity and dynamics of life, which carbon provides in spades.
Heaviness and Rarity in the Universe
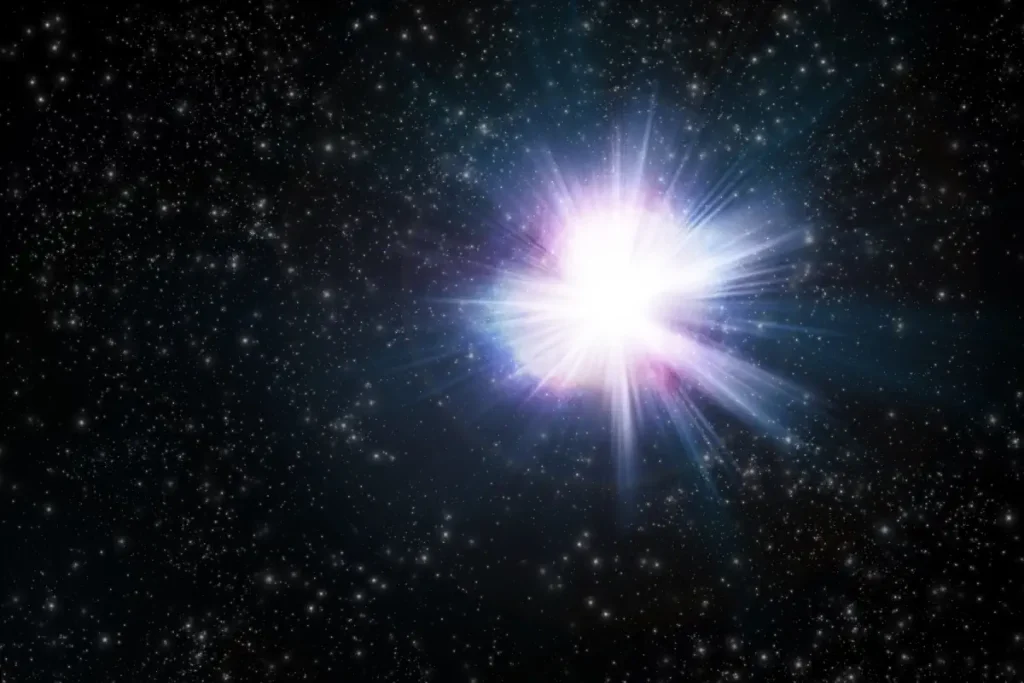
A fundamental principle in cosmic evolution is that heavier elements are rarer in the universe. This rarity is rooted in the processes of stellar nucleosynthesis. Stars, through their life cycles, primarily produce hydrogen and helium. Heavier elements, such as carbon, nitrogen, and oxygen, are formed in the later stages of a star’s life and spread into the universe during supernova explosions.
Carbon and oxygen were not created in the Big Bang, but rather much later in stars. All of the carbon and oxygen in all living things are made in the nuclear fusion reactors that we call stars. The early stars are massive and short-lived. They consume their hydrogen, helium, and lithium and produce heavier elements. When these stars die with a bang they spread the elements of life, carbon, and oxygen, throughout the universe. New stars condense and new planets form from these heavier elements. This sets the stage for life to begin.
As we move further down the periodic table, the processes required to create these heavier elements become less frequent and more complex. Consequently, the heavier the element, the rarer it tends to be in the universe.
While the universe is teeming with a variety of elements, it is the unique combination of carbon’s bonding versatility and its relative abundance in the cosmos that positions it as the elemental cornerstone of life.
5. Water Solubility
In the context of life as we know it, the solubility of compounds in water plays a pivotal role. Let’s delve deeper into the significance of water solubility when it comes to carbon and silicon compounds.
The Significance of Water in Biochemistry
Water is often referred to as the “universal solvent” due to its unique ability to dissolve a wide array of substances. On Earth, water forms the matrix in which nearly all biochemical reactions occur. Its polar nature allows it to interact with various molecules, facilitating their movement, interaction, and the subsequent reactions necessary for life processes.
Carbon Compounds and Their Affinity for Water
Carbon, with its versatile bonding capabilities, can form molecules that are both hydrophilic (water-loving) and hydrophobic (water-averse). This property is essential in crafting molecules with a range of functionalities. For instance, the hydrophilic heads and hydrophobic tails of phospholipids enable the formation of cellular membranes. The water solubility of many carbon-based molecules ensures that they can readily participate in biochemical reactions, be transported within organisms, and interact in the aqueous environments of cells.
The Limitations of Silicon in Aqueous Environments
While silicon shares some similarities with carbon, especially in its ability to form four bonds, its compounds often exhibit very different behaviors in water. Many silicon-based compounds are not soluble in water. This insolubility limits their potential participation in the dynamic, water-based chemistry of life. Silicon compounds, when interacting with water, tend to form silicates that precipitate out of solution. This means that if life were to be based on silicon, the central solvent (like water) would likely need to be different, and such a solvent might not support the myriad of chemical reactions required for life as we understand it.
The solubility contrast between carbon and silicon compounds in water offers a clear advantage to carbon when considering life’s chemistry on Earth. Water doesn’t merely act as a passive medium; it actively participates in and influences biochemical reactions. The fact that so many carbon compounds can interact harmoniously in water, facilitating complex chains of reactions, emphasizes carbon’s superior suitability as life’s foundational element in Earth’s aqueous environment.
In essence, while silicon has some chemical properties that might hint at its potential in a hypothetical life form, its limited interaction with water, a key component of life on Earth-poses significant challenges. Carbon, with its ability to readily dissolve and interact in water, stands out as the more viable element to support the intricate web of life processes.
6. Lightweight Element
When contemplating the elements and conditions that might be essential for the genesis and evolution of life, the physical characteristics of those elements become just as crucial as their chemical properties. Carbon’s relatively lightweight nature is one such attribute that appears to have had a profound influence, especially in the early epochs of life’s history on Earth. Here’s a deeper exploration of the subject:
Setting the Scene: Earth’s Primitive Atmosphere
In the primordial stages of our planet, the atmosphere was vastly different from what it is today. Volcanic eruptions released gases, and various molecules floated around, awaiting the right conditions and combinations to kickstart life’s intricate processes. The ability for molecules to stay suspended in the atmosphere for extended periods would have been a significant advantage, allowing them more opportunities to interact and combine with other molecules.
Carbon’s Advantage in the Aerial Dance
Being a lightweight element, carbon can form molecules that are buoyant enough to remain airborne. This buoyancy ensures that carbon-based molecules have extended interaction times in the atmosphere. Such interactions could lead to the creation of more complex molecules by capturing energy from sources like ultraviolet radiation from the sun. Think of carbon molecules as dancers in the atmospheric ballroom, staying afloat longer, engaging with multiple partners, and, in doing so, orchestrating the preliminary steps of life’s waltz.
Implications for the Emergence of Life
The early atmosphere likely acted as a vast laboratory, with myriad chemical reactions occurring simultaneously. Carbon’s ability to float meant that its compounds had a greater chance of participating in these reactions, increasing the likelihood of forming complex organic molecules. Some theories even suggest that the synthesis of simple organic molecules, possibly even amino acids, could have taken place in the atmosphere before they rained down onto the Earth’s surface.
Contrast with Heavier Elements
Heavier elements, due to their greater mass, would have had a harder time remaining suspended in the atmosphere. Their compounds would settle more quickly, reducing the time and opportunity for aerial chemical interactions. Carbon’s lightweight nature, in contrast, gave it a sort of “extended playground” in the sky, increasing its chances of engaging in life-sparking reactions.
In summary, the lightweight characteristic of carbon isn’t just a minor footnote in its resume. It might have been one of the pivotal reasons carbon played a starring role in the story of life’s origin, offering it an atmospheric arena to forge the initial pathways to life as we know it.
Sources
- Header image: “Elk in Forest” on Deposit Photos
- “Understanding the Evolution of Life in the Universe” on the NASA website
- Carbon on Wikipedia
- Silicon on Wikipedia
- Moon Landings: All-Time List [1966-2025] - February 2, 2025
- What Is Max-Q and Why Is It Important During Rocket Launches? - January 16, 2025
- Top 10 Tallest Rockets Ever Launched [2025 Update] - January 16, 2025