An essential step towards a carbon-neutral future could be reached through dispersed power grids, featuring networks of local-scale renewable energy and battery storage plants. To prevent these power grids from damaging themselves and their surroundings when electrical faults arise, they must be integrated with “circuit breakers”, which temporarily interrupt the current flowing through them.
However, currently available circuit breakers cannot handle the medium-voltage direct currents best suited for these grids. Through an innovative new circuit breaker design, Steve Schmalz and his colleagues at Eaton Corporation, the Illinois Institute of Technology (IIT), Virginia Tech, and the National Energy Technology Laboratory (NETL), hope that this challenge will soon be overcome.
AC vs DC
Currents in electrical circuits can flow in two distinct ways: either periodically changing direction in alternating current (AC), or maintaining a constant direction in direct current (DC). Each of these types has its own advantages. Owing to its high efficiency and low maintenance costs, AC has dominated the US power grid for over a century. However, through the latest advances in semiconductor electronics, DC is now becoming increasingly popular.
With its ability to transmit large amounts of power with very little energy loss, DC is now the current of choice in a wide array of applications, which vary depending on voltage. While low-voltage DC is commonly used in consumer electronics, LED lighting, and electric vehicles, high-voltage DC can reliably transmit power across large distances – making it key to the performance of undersea and underground cables.
While the technology required to implement DC at low and high voltages is now maturing, there remains a gap in the middle, spanning voltages ranging from 1 to 100 kilovolts. Such “medium-voltage DC” is still in the early stages of technological development.
Advantages of DC over AC
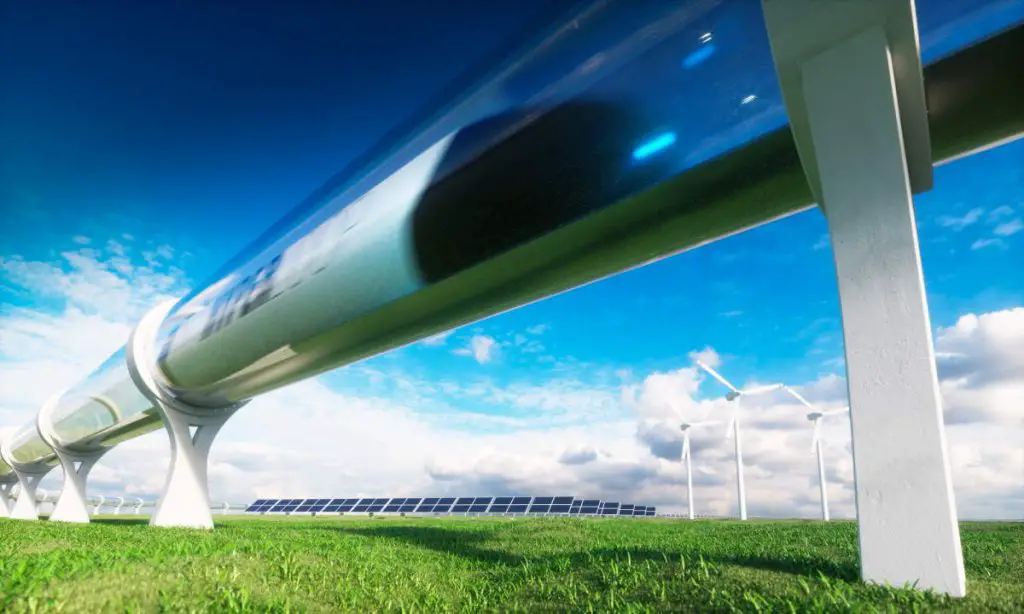
Direct Current (DC) has many advantages over Alternative Current (AC). Some of them are:
- Energy efficiency: DC power is significantly more energy efficient than AC power. DC motors and appliances have higher efficiency and power-to-size characteristics. Another example of DC’s efficiency is, DC-based lighting (LED) is as much as 75% more efficient than incandescent lighting (incandescent light is the classic light bulb).
- Less energy loss during transfer: The greater efficiency of DC also means less energy loss during transfer. This enables more efficient electricity delivery over long distances.
- Compatibility with renewable energy sources: DC is inherently compatible with renewable energy sources such as solar and wind. These sources generate power intermittently, they do not produce power during the night or when the wind stops. So, in order to deliver a reliable power supply, renewable energy sources should be integrated with storage facilities in most applications. These batteries need DC electricity.
- Electronic equipment operates on DC power: To use renewables, we first produce energy (DC), then store it (DC), then convert it to AC in order to transfer it, and then convert it to DC again to supply electronic equipment. There is a loss of 5% to 20% when AC power is converted to DC power (or vice versa). If we could eliminate these DC/AC and again AC/DC conversions, our power grids would be 10%-40% more efficient.
- Residential loads use DC: Many residential loads are DC-operated, i.e. microwave ovens, computers, laptops, smartphones, lighting, and electric vehicles (cars, e-bikes, e-scooters, etc).
- DC does not create reactive wastes (inductive and capacitive): that influence, in particular, long-distance applications, and this enables power distribution employing underground wires. This would be impractical with the AC form due to the capacitive wastes.
- With DC, there is no skin effect problem: When DC current flows through the conductor it gets distributed uniformly throughout the cross-section area of the conductor or skin. However, an alternating current flowing through the conductor does not distribute uniformly. It concentrates near-surface area of the conductor. this phenomenon is known as the skin effect. Because of this phenomenon, the effective cross-section area of the conductor is reduced, hence the effective resistance of the conductor increases, which means energy losses.
- Data Centers use DC: Today, the most remarkable new consumers of electric energy are the big IT companies, like Apple, Google, Visa, etc. They use huge data centers and server farms that use a great amount of electricity. They require DC form as electronics basically require DC sources.
- DC is the future: Modern cities and villages are being designed in Europe and China and these futuristic cities will be totally DC-powered.
Dispersed Power Grids
Steve Schmalz and his colleagues at Eaton Corporation, alongside partners at IIT, Virginia Tech, and NETL, recognize two key advantages of medium-voltage DC: it is both the most practical voltage range for converting AC into DC and is well suited to transmitting power across local-scale regions.
Because of these advantages, progress in medium-voltage DC technology could bring immense benefits to the development of dispersed power grids. Rather than being generated on industrial scales in single locations, such as coal or nuclear power plants, energy in these grids is produced, stored, and distributed on local scales.
In a dispersed power grid, the excess energy produced by networks of smaller renewable energy plants, including wind and solar farms, can easily be stored in local battery facilities and then released when required. For example, a solar farm may produce more energy than demand requires during the day – and so this excess can be used to charge a battery. This energy can then be used to power homes and businesses after sunset when no solar power is being generated.
On top of this, the use of medium-voltage DC would mean that these new systems could easily plug into existing AC power grids, without major changes to infrastructure.
“For example, a medium-voltage DC system could serve as a bi-directional link between separate AC grids that are unsynchronised and could otherwise not be connected without massive reconfiguration or replanning,” explains Schmalz.
“This could provide added grid resilience allowing one AC grid with excess capacity to share with an adjacent grid that is being overtaxed. Depending on the geography, retrofitting medium-voltage power converters between the AC and DC systems could control the power flow between grids with less added infrastructure, and minimize the need to clear rights-of-way for completely new AC power lines.”
Altogether, the efficiency and flexibility offered by dispersed, small-scale power grids could make them central to the widespread rollout of renewable energy. As such, dispersed power grids could be essential in drastically reducing greenhouse gas emissions. However, before this can be achieved, new innovations in the technology required to support medium-voltage DC will be essential.
Interrupting Faulty Circuits using Circuit Breakers
Currently, one of the biggest roadblocks in this development is the need for an essential safety component, named a circuit breaker. These devices come in a wide array of designs, but all operate by the same general principles.
During electrical faults, which can produce damaging or even dangerous surges in current, powered sensing devices detect any excess flows of current by picking up the heating or magnetic effects they generate.
Once the fault is detected, the device opens a switch to break the circuit, temporarily interrupting the path of its flowing current, thus stopping the surge. In mechanical circuit breakers, this involves using transducers in response to the excess current to trigger switch mechanisms such as springs or levers – physically separating two contacts in the circuit in a controlled way.
This separation generates an arc of electricity where the current jumps between the contacts, which must be cooled, contained, and extinguished as quickly as possible.
The main advantage of mechanical circuit breakers is that they allow currents to flow with very little conduction loss in normal circumstances. However, since their contact separation mechanisms can take a long time to trigger, the level of safety they offer can be limited. In addition, the emergence of an arc can damage the device, limiting its operational lifetime.
More fundamentally, quenching an arc between parting contacts is more difficult to accomplish in DC, as the current does not periodically reach zero like in AC, allowing the arc to extinguish. So far, these drawbacks have meant the use of mechanical circuit breakers has largely been limited to AC and low-voltage DC systems. These challenges make upscaling to medium-voltage DC more difficult.
Alternatively, solid-state circuit breakers involve the use of semiconductor-based transistors, which interrupt flowing currents within the semiconductor material itself. These switches don’t generate arcs and can be switched to block current far more quickly when electrical faults arise. This makes them ideal for applications where circuit breakers are placed close to the equipment being protected, such as battery packs in electric vehicles.
However, solid-state circuit breakers also induce high energy losses. This means that much of the current flowing through them is lost as heat – creating the need for separate cooling systems. Currently, it would be incredibly difficult to scale this setup to medium-voltage levels, without sacrificing their cost and efficiency.
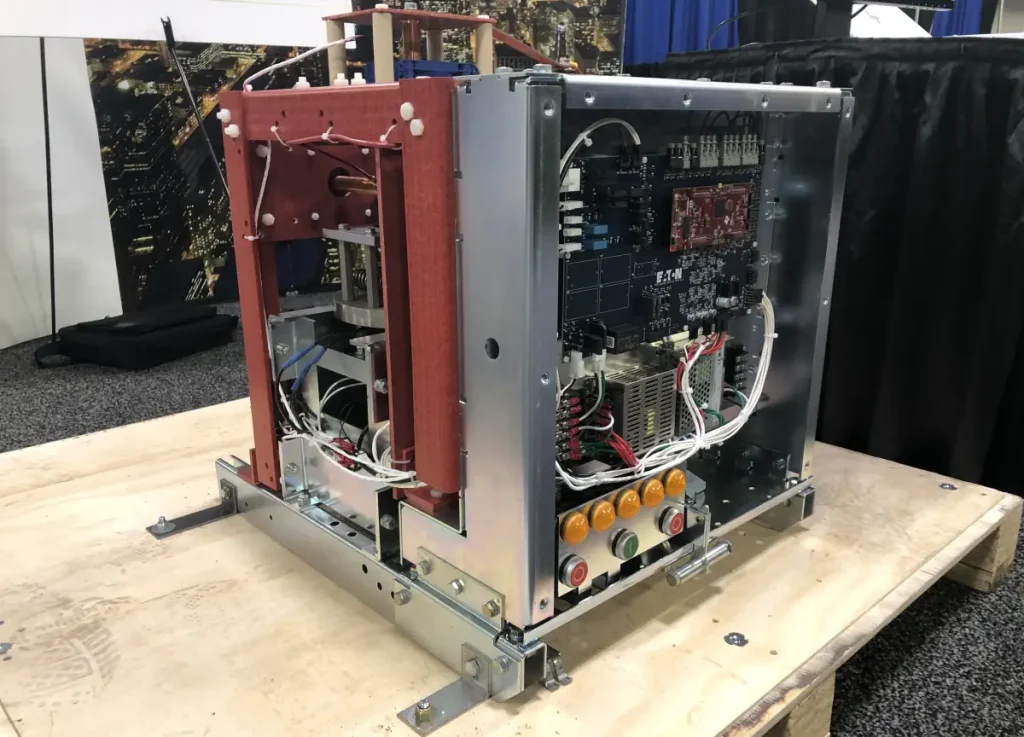
Hybrid Circuit Breakers
One further design, named a ‘hybrid’ circuit breaker combines the advantages of mechanical and solid-state circuit breakers – rapidly interrupting circuits when faults arise, while keeping power losses comparatively low.
Hybrid circuit breakers can have different configurations, but each one employs four basic functional elements: a mechanical switch, at least one semiconductor-based switch which ultimately interrupts the current flow, a means of transferring or ‘commutating’ current flow from the mechanical switch to the semiconductor interrupter, and a system of surge arrestors that limit the peak voltage and dissipate any excess electrical energy as heat.
When a fault occurs, the commutator transfers the current to the semiconductor switch. The mechanical switch then opens, but only once the current has been completely diverted – preventing an arc from forming. Afterwards, the semiconductor is turned off, and any remaining current is dissipated by the surge arresters.
Roadblocks to Commercial Rollout
In principle, this combination of circuit breaking mechanisms makes hybrid circuit breakers ideal for integrating with medium-voltage DC systems. However, there are still challenges to overcome. While existing hybrid circuit breaker designs provide lower energy losses than solid-state circuit breakers, they don’t typically provide the same rapid response times because they are limited by the speed of the mechanical switches. Meanwhile, the method of commutation may compromise the overall efficiency that the hybrid circuit breaker can truly achieve.
Prototypes for medium-voltage DC circuit breakers are now in development by several research teams around the world, and are now capable of handling voltages ranging from 7 to 12 kilovolts. However, these challenges mean that they have only been demonstrated in lab settings and aren’t yet considered commercially-viable products.
Designing an Improved Hybrid Topology
Through their research, Schmalz and his colleagues have made promising steps towards overcoming this barrier, through the development of a modular, scalable device, specifically designed for interrupting medium-voltage DC current, with key improvements to the essential hybrid breaker elements.
Some previous hybrid designs employ methods to transfer current from the mechanical switch to the semiconductor interrupter. Such designs require the insertion of another semiconductor in the conduction path with the mechanical switch, which compromises overall efficiency.
In the team’s new design, current flows only through a closed mechanical switch under normal circumstances, minimizing energy loss. This switch is connected in parallel to a second conduction path, containing a novel power electronic circuit that can carefully track the current flowing through it, and subsequently inject current in the opposite direction.
When a fault occurs, this injector transfers the current from the mechanical switch to its own conduction path – which also contains the semiconductor interrupting switch. When the current passing through the mechanical switch reaches zero, it opens with no arcing or damage to the contacts.
Other key improvements in the design reside in the mechanical switch, which employs contacts enclosed in a vacuum and a Thomson coil-based actuator capable of forcing the contacts open 20 times faster than traditional mechanisms.
The vacuum provides higher-voltage insulating properties at shorter contact separation distances. These traits combine to dramatically improve the response time to be comparable to that of pure solid-state circuit breakers, because the semiconductor interrupting switch can be switched off soon after the contacts part, completing the fault interruption process.
Another key advantage of this design is that the semiconductor interrupting switch is completely modular – meaning any number of them could be connected in series to scale to higher voltage systems. In turn, this means that the surging current can be shared between as many interrupters as required.
Such an easily scalable setup allowed Schmalz’s team to optimize their system over a wide range of voltages. In their setup, they used their hybrid circuit breaker to handle up to 6 kilovolts.
Compared with previous designs, this setup offered both comparatively fast response times, improved efficiency, and far higher power density. This allowed it to be compacted into far smaller volumes, removing any need for bulky and expensive cooling apparatus.
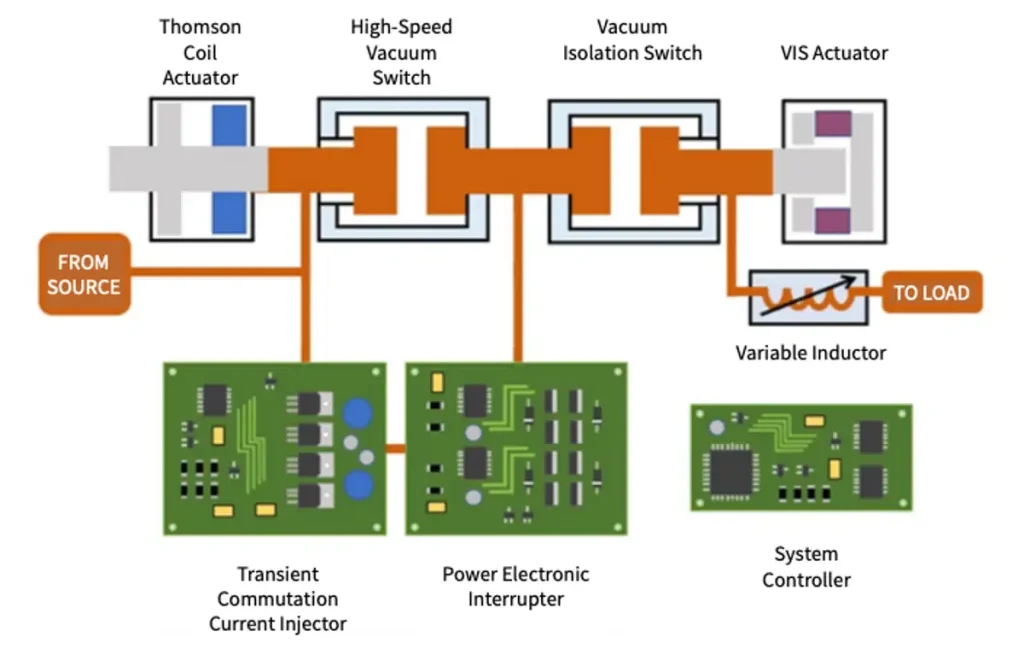
Bringing Medium-Voltage DC to Maturity
Schmalz and his colleagues hope that their novel hybrid circuit breaker design could help to overcome many of the challenges currently faced by medium-voltage DC technology. By integrating their device into power grids, operators could confidently safeguard their systems against damage when electrical faults arise.
If the device becomes commercially available in the near future, it will enable easier integration of renewable energy and battery storage into existing power grids, in addition to more efficient powering of native DC loads that connect to power grids, such as railways and data centers.
By implementing dispersed power grids, capable of generating and delivering energy on local scales, Schmalz and his colleagues hope that an important step toward a carbon-neutral future may finally be achieved.
Meet the researcher
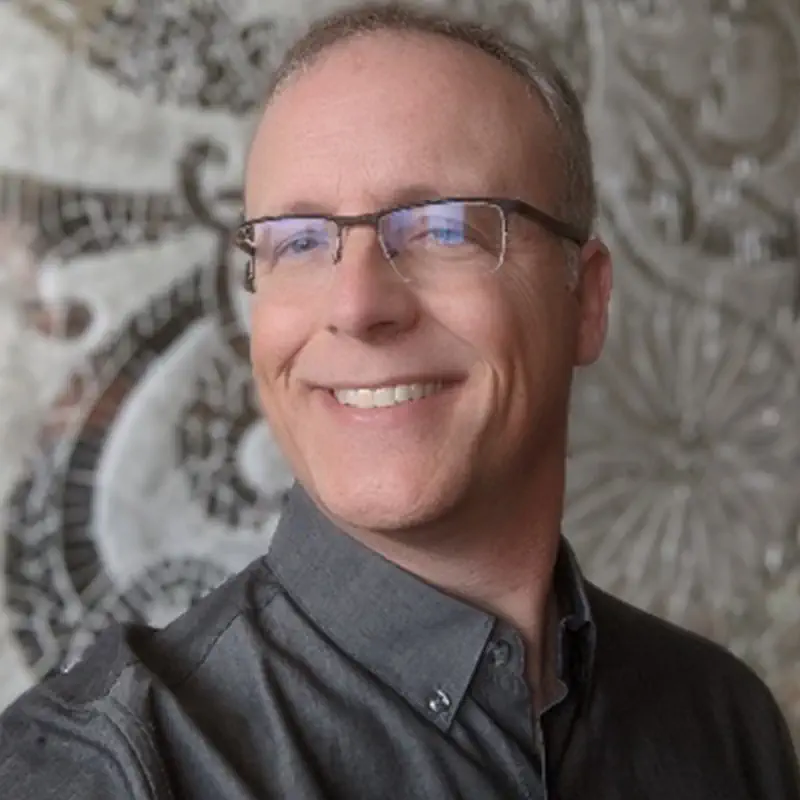
Steven Schmalz earned both his Bachelor’s and Master’s degrees in Electrical Engineering from Marquette University.
He has worked at Eaton Corporation Research Labs for over 30 years, where he is now an Engineering Senior Specialist.
His career has spanned a wide range of research interests – from sensing technologies to energy management and methods for protecting electrical systems.
Schmalz has served as the Principal Investigator on several research projects funded by the U.S. government, designing technology for both ground-based and aerospace power applications.
He is also a member of the Institute of Electrical and Electronics Engineers, is a licensed Professional Engineer in the State of Wisconsin, and is also a Certified Eaton Design For Six Sigma (DFSS) Green Belt.
Contact
- E-mail: SteveSchmalz@eaton.com
- Web: eaton.com
Key Collaborators
- Dr. Zheng (John) Shen, Simon Fraser University
- Dr. Dong Dong, Virginia Tech
- Mr Steven Chen, Eaton Power Components Division
- Dr. Richard Byron Beddingfield, North Carolina State University (via the National Energy Technology Laboratory)
- Dr. Andrew Rockhill, Eaton Research Labs
- Dr. Ian Brown, Illinois Institute of Technology
- Mr. Bradford Irons, Eaton Power Components Division
Funding
The information, data, or work presented herein was funded in part by the Advanced Research Projects Agency-Energy (ARPA-E), U.S. Department of Energy, under Award Number DE-AR0001111. The views and opinions of authors expressed herein do not necessarily state or reflect those of the United States Government or any agency thereof.
Further reading
- Y Zhou, Y Feng, N Shatalov, R Na, ZJ Shen, An ultraefficient DC hybrid circuit breaker architecture based on transient commutation current injection, IEEE Journal of Emerging and Selected Topics in Power Electronics, 2020, 9, 2500. https://doi.org/10.1109/JESTPE.2020.2983354
- B Lequesne, T Holp, S Schmalz, M Slepian, H Wang, Frequency-Domain Analysis and Design of Thomson-Coil Actuators, In 2021 IEEE Energy Conversion Congress and Exposition (ECCE), 2021, 4081. https://doi.org/10.1109/ECCE47101.2021.9596019
- J Liu, L Ravi, C Buttay, R Burgos, S Schmalz, A Schroedermeier, ZJ Shen, D Dong, 12 kV 1 kA Breaking Capable Modular Power Electronic Interrupter with Staged Turn-off Strategy for Medium Voltage DC Hybrid Circuit Breaker, IEEE Transactions on Industry Applications, 2022. https://doi.org/10.1109/TIA.2022.3185570
Sources
- This article originally appeared in Scientia, with the title “Steve Schmalz | An Innovative Circuit Breaker for Renewable Power Grids”. Read the original article.
- “Advantages of DC over AC in Power Plants” on the Linquip Tech News website
- 10 Advantages of Direct Current (DC) on the Electrical Terminology website
- “9 Reasons Why DC May Replace AC” by Gregory Reed on the Electrical Industry News Week website
- “What is Skin Effect? Definition Factors affecting” on the Electric Portal website
- An Innovative Medium-Voltage DC Circuit Breaker for Renewable Power Grids - October 13, 2022
- Scientists are Mapping the Earth’s Magnetosphere with Energetic Atoms - September 30, 2022
- Scientists are Developing Recyclable and Self-healing Plastics - August 9, 2022
One reply on “An Innovative Medium-Voltage DC Circuit Breaker for Renewable Power Grids”
an interesting concept for the MVDC breaker design and constructure, if possible, let’s continue further discussion esp. about the industrialization and commercialization.