A significant proportion of the world’s population has little to no access to clean water, and the water consumed by industrial activities continues to grow. Researchers from the Nanotechnology Enabled Water Treatment (NEWT) Center, which is headquartered at Rice University, are developing cutting-edge water purification technologies that can provide communities with access to clean and safe drinking water. They are also creating new wastewater treatment methods that allow the reuse of industrial effluent, to minimize freshwater withdrawals by industries. Instead of conventional methods that use large amounts of chemicals and energy, NEWT technologies are chemical-free, and often utilize solar energy.
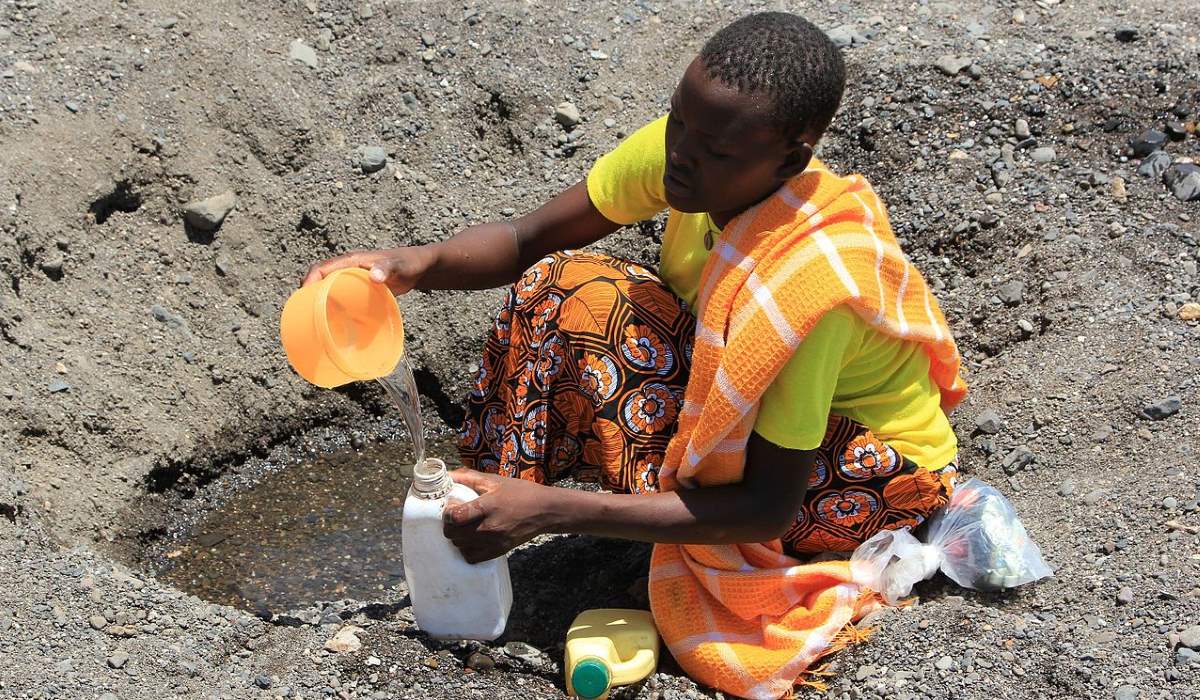
Related: World’s Water Inequality Crisis
Responding to Water Shortages
Many people across the globe do not have access to clean water, and there remains a vital need to provide communities with potable water supplies. Seawater and other saline or compromised water sources are often readily available, but these supplies are typically unsuitable for consumption, given their high concentrations of salts, microbes, toxic metals, and other contaminants. Such water sources need to be purified before they can be used.
Multiple research teams from the “Nanotechnology Enabled Water Treatment” (NEWT) Center are working to tackle these urgent problems. Funded by the US National Science Foundation, the Center is made up of researchers from Rice University, Arizona State University, Yale University, and the University of Texas at El Paso, with expertise spanning diverse disciplines, including environmental engineering, chemical engineering, materials science, chemistry, and physics. NEWT’s vision is to enable access to water of suitable quality almost anywhere in the world by developing next-generation, easy-to-deploy modular treatment systems enabled by nanotechnology.
Membrane Distillation
One water purification method that NEWT researchers are working to improve is called “membrane distillation”. In the process of membrane distillation, hot saltwater is passed over a membrane on one side, while cold, freshwater flows on the other side of the membrane (see notes 1). The membrane is porous but keeps the saltwater and freshwater streams apart. The temperature difference across the membrane and between the two streams causes hot water from the saltwater side to vaporise through the membrane and join with the colder freshwater stream. The process does not require the use of chemicals and, overall, separates water from saltwater solutions.
The migration of water vapour from the saltwater side to the freshwater side occurs to a greater extent when the difference in temperature across the membrane is higher. When the temperature of the saltwater is high, the extent to which vaporisation occurs is similarly high. Likewise, when the freshwater temperature is low, the extent to which condensation occurs is also high.
These factors do, however, reveal an inherent limitation with membrane distillation. The act of vaporization lowers the temperature of the hot, saltwater side, and condensation increases the temperature of the cold, freshwater side. The membrane also transfers some of the heat energy from the saltwater side to the freshwater side. These and other temperature-related factors subsequently reduce the difference in temperature across the membrane, therefore lowering the volume of water that can migrate across it. To maximize water vapor migration, one would need to maintain a large temperature difference across the membrane.
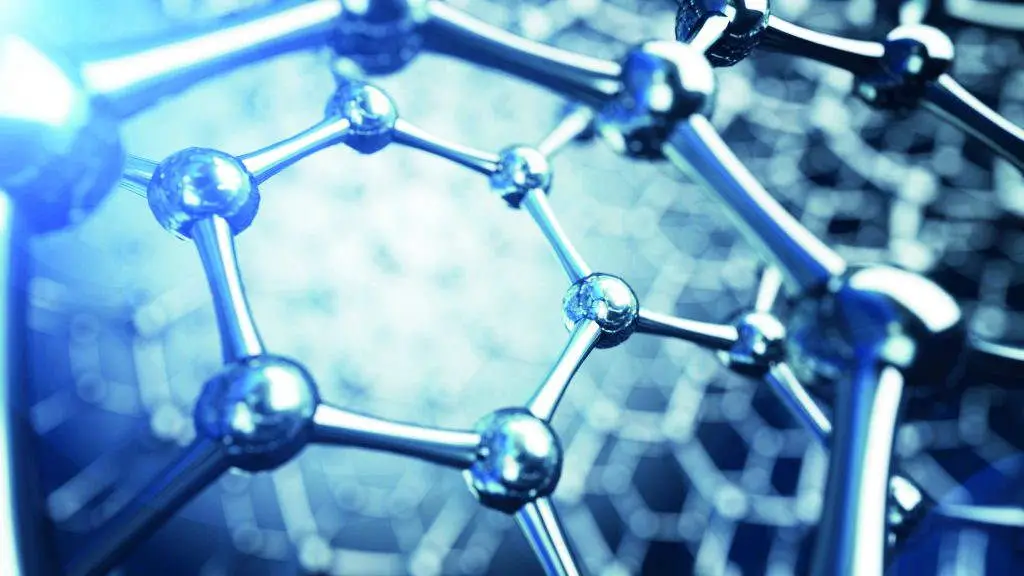
Sustainable Water Purification: Harnessing the Sun’s Energy
Maintaining the temperature difference can be achieved by heating the membrane itself. Researchers from the NEWT Center found that by adding a layer that can absorb solar energy to the membrane surface on the saltwater side, and then directing sunlight to this layer, they were able to increase the quantity of water vapor transferred. The team’s new technology, referred to as “nanophotonics enabled solar membrane distillation” (NESMD), can thus be powered locally, using free, clean, and widely available energy from the Sun.
In the team’s NESMD technology, the layer on the membrane that absorbs sunlight is called “carbon black” – a substance similar to activated charcoal. Sunlight is directed through an array of lenses to the carbon black layer, which absorbs nearly all frequencies of visible light, transferring the energy as heat to the membrane.
NESMD is relatively cheap to operate since any potential water purification plant would not need to heat the entire volume of saltwater. The NEWT researchers hope that their environmentally friendly purification process can be applied at different scales – from whole communities down to individual households, many of whom will not be connected to the national grid.
The team also investigated other energy-absorbing materials for NESMD. In one study, they applied “silica”, the main constituent of sand and glass, covered with gold to the surface of the membrane. The researchers found that the carbon black materials absorb more of the high-energy radiation in sunlight, comprising blue and violet light, whereas the silica-gold materials absorb more of the low energy radiation, corresponding to red and yellow light. Both NESMD membrane compositions performed more effectively than conventional membranes, maintaining the temperature difference needed to prolong vaporization.
For applications that require large volumes of water 24 hours a day, such as industrial applications, NEWT researchers have also developed “dual-power membranes”. These membranes harness energy directly from sunlight when available and then operate on electricity at night. One team of NEWT researchers developed a dual-power membrane comprising a nanolayer of boron nitride on a stainless-steel wire cloth, which demonstrates superior performance, making it suitable for industrial applications.
Another group of researchers fabricated a dense, non-porous “pervaporation” membrane from a polymer called Nexar™. The team found that their membrane exhibited a salt separation performance superior to commercial pervaporation membranes, and equivalent to that of commercial membrane distillation membranes.
In another study, NEWT scientists used egg-shell waste to develop a highly efficient dual-power membrane. Specifically, they used the egg membrane, found just inside the shell, whose porous structure efficiently allows the selective movement of water into and out of the egg during incubation and hatching. In the manufacture of egg-containing products, these membranes are often discarded along with the shells. Therefore, combined with the use of renewable energy from the sun, the team’s technology represents an even more environmentally friendly and sustainable solution to water purification.
Selective Removal of Ions
In addition to their efforts to remove common salts from water, NEWT researchers have also been working on methods that remove contaminant ions that are far less abundant than common salts. For example, nitrate and chromate are negatively charged ions that cause a range of human health problems. Sulfate and calcium ions, although non-toxic at typical concentrations, can form solid deposits that cover surfaces and cause a range of problems in homes and industrial processes. Removal of these ions is challenging because highly abundant common salt ions interfere with the treatment processes.
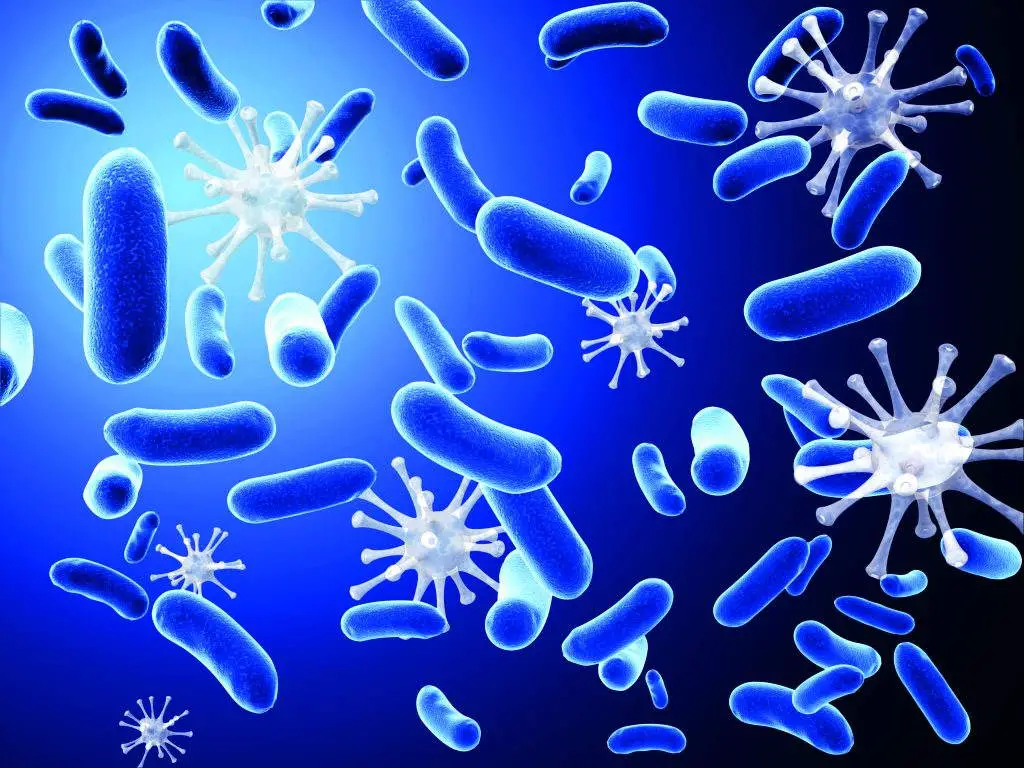
Most techniques for removing calcium involve chemical treatments. Thus, NEWT researchers wanted to develop an alternative method that can selectively remove calcium ions from water using a process known as “electrosorption”. During electrosorption, ions that have an opposite charge to that of an electrode are attracted to the electrode, removing these ions from water.
Then, the charge on the electrode is reversed, releasing the ions into a saltwater stream that will be disposed of. The research team developed nanocoatings. When applied to the electrode surface, they allow calcium to approach the electrode faster than the interfering common ions. This makes the electrode surface highly selective to calcium ions and provides a very effective method of calcium ion removal.
In several other studies, NEWT research teams developed electrodes that can remove negatively charged ions, including sulfate. Some salts of sulfate ions are very insoluble in water, and over time they can contribute to water scaling. At higher concentrations, sulfate ions can even be converted into hydrogen sulfide by bacteria.
Hydrogen sulfide is a toxic gas and its production is particularly problematic in the oil and gas industries, where sulfate and bacteria are abundant in the water used during crude oil extraction and processing. The researchers demonstrated effective and selective removal of sulfate ions from water using novel nano-electrode materials in the electrosorption process.
Using a similar approach, NEWT scientists also conducted research on the selective removal of a negatively charged ion called chromate. This highly toxic chemical can cause cancer and is a widely occurring contaminant in groundwater. In their study, the researchers created an electrode made from a type of graphene called “reduced graphene oxide”, upon which they grew nanocrystals of a cobalt-containing compound.
Applying a positive voltage to the conductive graphene material allowed it to attract the negatively charged chromate ions, while the nanocrystals could then efficiently trap the ions in place. By then applying a negative voltage to the electrode, the researchers were able to release the chromate into a saltwater stream for disposal.
Deactivation of Microbes
The chemical-free deactivation of microbes in water is another important part of NEWT’s research portfolio. Boiling large volumes of water to kill bacteria is considered inefficient. Instead, the team developed a method of successfully disinfecting water that uses gold nanorods or carbon black to channel solar energy into the water. This results in localized heating of the water, which subsequently kills nearby microbes.
Following a different approach, another team of NEWT researchers designed UV-light side-emitting optical fibers that can disinfect flowing water and prevent the growth of biofilms on surfaces, which would otherwise harbor pathogens such as Legionella. By depositing a layer of silica nanoparticles onto the surface of each optical fiber, the researchers could cause the germicidal UV light to become scattered from the side of the fiber like a glowstick. Upon testing their technology on E. Coli and other bacteria, the team was able to effectively kill over 99% of the bacteria in water samples and in slimy biofilms growing on surfaces.
Multidisciplinary Nanotechnology Research
Evidently, the work performed by the NEWT researchers is very comprehensive. Their collaborative and multidisciplinary efforts will prove vital to the health and wellbeing of communities and individuals across the world who do not have access to clean water, particularly as climate change and a growing human population continue to place greater stress on our global water supplies.
This article originally appeared in Scientia, with the title of “Developing Sustainable Water Purification Technologies”.
The NEWT Center
This research was carried out by academic members of the Nanotechnology Enabled Water Treatment Center, NEWT, a collaboration of research teams from across the US, with headquarters at Rice University. The principal investigators of the team are Professor Pedro Alvarez, Professor Qilin Li, and Professor Naomi Halas from Rice University, Professor Menachem Elimelech from Yale University, and Professor Paul Westerhoff from Arizona State University.
In addition to their research partnerships with universities and industries across the world, the NEWT Center is also committed to providing high school students and teachers and undergraduates, as well as postgraduates with hands-on research experiences.
Contact
- Nanotechnology-Enabled Water Treatment (NEWT) Center, Rice University, Houston, TX, USA
- Email: info@newtcenter.org
- Web: https://newtcenter.org/
Funding
US National Science Foundation
Further reading
- ER Thomas, A Jain, SC Man, Y Yang, MD Green, WS Walker, F Perreault, ML Lind, R Verduzco, Freestanding self-assembled sulfonated pentablock terpolymer membranes for high flux pervaporation desalination, Journal of Membrane Science, 2020, 613, 118460.
- K Zuo, X Huang, X Liu, EM Gil Garcia, J Kim, A Jain, L Chen, P Liang, A Zepeda, R Verduzco, J Lou, and Q Li, A Hybrid Metal−Organic Framework−Reduced Graphene Oxide Nanomaterial for Selective Removal of Chromate from Water in an Electrochemical Process, Environmental Science & Technology, 2020, 54, 20, 13322.
- M Lanzarini-Lopes, B Cruz, S Garcia-Segura, A Alum, M Abbaszadegan, P Westerhoff, Nanoparticle and Transparent Polymer Coatings Enable UV-C Side-Emission Optical Fibers for Inactivation of Escherichia coli in Water, Environmental Science & Technology, 2019, 53, 10880.
- PD Dongare, A Alabastri, O Neumann, P Nordlander, NJ Halas, Solar thermal desalination as a nonlinear optical process, PNAS, 2019, 116, 27.
- J Kim, A Jai, K Zuo, R Verduzco, S Walker, M Elimelech, Z Zhang, X Zhang, Q Li, Removal of calcium ions from water by selective electrosorption using target-ion specific nanocomposite electrode, Water Research, 2019, 160, 445.
- K Zuo, J Kim, A Jain, T Wang, R Verduzco, M Long, Q Li, Novel Composite Electrodes for Selective Removal of Sulfate by the Capacitive Deionization Process, Environmental Science & Technology, 2018, 52, 9486.
- PJJ Alvarez, C Chan, M Elimelech, N Halas, and D Villagran, Emerging opportunities for nanotechnology to enhance water security, Nature Nano, 2018, 13, 634.
- S Loeb, C Li, J-H Kim, Solar Photothermal Disinfection using Broadband-Light Absorbing Gold Nanoparticles and Carbon Black, Environmental Science & Technology, 2018, 52, 205.
- PD Dongare, A Alabastri, S Pedersen, KR Zodrow, NJ Hogan, O Neumann, J Wu, T Wang, A Deshmukh, M Elimelech, Q Li, P Nordlander, NJ Halas, Nanophotonics-enabled solar membrane distillation for off-grid water purification, PNAS, 2017, 114, 27.
- J Wu, KR Zodrow, PB Szemraj, Q Li, Photothermal nanocomposite membranes for direct solar membrane distillation, Journal of Materials Chemistry A, 2017, 5, 23712.
Notes
- A membrane is a selective barrier that allows some things to pass through but stops others. There are biological and synthetic membranes.
- Biological membranes include
- cell membranes (outer coverings of cells or organelles that allow passage of certain constituents)
- nuclear membranes which cover a cell nucleus
- tissue membranes, such as mucosae and serosae.
- Synthetic membranes are made by humans for use in laboratories and industry (such as chemical plants).
- Biological membranes include
- An Innovative Medium-Voltage DC Circuit Breaker for Renewable Power Grids - October 13, 2022
- Scientists are mapping the Earth’s Magnetosphere with Energetic Atoms - September 30, 2022
- Scientists are Developing Recyclable and Self-healing Plastics - August 9, 2022