Quasicrystals are among the newest and most exciting discoveries in the wider field of materials physics – but to date, many aspects of their exotic physical properties remain entirely unexplored. Since soon after their initial discovery, Dr. Zbigniew Stadnik at the University of Ottawa has made important contributions to our understanding of quasicrystals, including their magnetic and electronic characteristics. Building on his decades of experience in the field, he now hopes to gain a complete understanding of the fundamental properties of these materials – potentially opening up a broad new range of real-world applications.
Quasicrystals
In condensed matter physics, researchers are constantly making discoveries that challenge our conventional understanding of how materials behave, and the molecular mechanisms responsible for their unique characteristics. In 1984, a particularly exciting new family of materials emerged. Named quasicrystals, these materials have crystal structures that can be easily defined mathematically – but all the same, are drastically different from those of regular crystals.
Within the crystal lattices of many solids, atomic arrangements can be found that reliably repeat on local scales. This means that if the lattice is moved along a straight line in any direction, it will eventually appear identical to its initial state – a property that mathematicians call “translational symmetry”. In contrast, quasicrystals feature no translational symmetry; instead, repetition emerges through their “rotational” symmetry, which can be seen when the structures are rotated about certain points.
This geometry was famously visualized by English mathematician Roger Penrose, who showed how 2D tiles in just two particular shapes can perfectly fit together, in arrangements extending out to infinity. Within Penrose’s pattern, any finite patch of tiles is never once repeated. Nonetheless, it displays a five-fold rotational symmetry, meaning the same infinite pattern will emerge five separate times when rotated completely about a single point.
In quasicrystals, these patterns show up in x-ray diffraction studies, where x-rays become scattered by thin sections of the crystals as they pass through. Using x-ray diffraction, physicists have shown that depending on their chemical composition, quasicrystals can display a seemingly endless variety of non-repeating crystal structures. With such a rich scope for future research, there is still much for physicists to learn about the physical characteristics of these exotic materials.
Uncovering Novel Properties
Despite their immense interest to physicists and mathematicians alike, quasicrystals can’t simply be studied out of curiosity for their crystal structures. An important part of their research is to determine whether they have interesting physical properties that widely differ from those of regular crystals – and if so, whether these properties can be readily exploited in new technological applications.
Already, the ways in which quasicrystals transport electrical currents and generate magnetic fields have been shown in experiments to be highly unusual. In parallel with these studies, condensed matter theories have been constructed to explore how these properties will vary in different scenarios – including extreme temperatures, and different chemical compositions.
Through a series of studies now spanning 25 years, Dr. Zbigniew Stadnik of the University of Ottawa has used the latest experimental techniques to investigate the mysteries of quasicrystals in detail and to assess whether existing theories can provide an accurate picture of how quasicrystals behave on atomic scales.
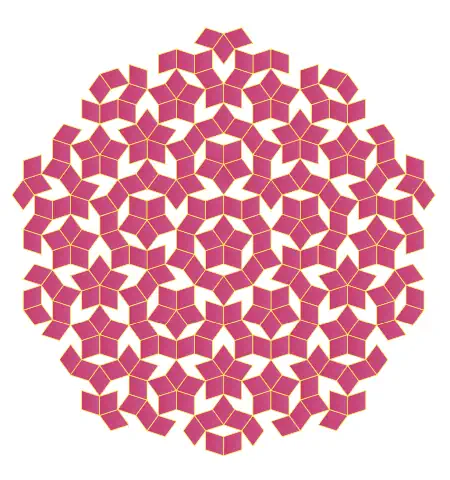
Abruptly Altered Resistivity
One particularly striking electrical property can be found in quasicrystals composed of metal alloys, whose crystal structures have a five-fold rotational symmetry. When cooled to just a few degrees above absolute zero, these materials can suddenly become extremely resistant to electrical currents. This ‘resistivity’ quickly increases as the temperature is further reduced – a property not found in metals.
Furthermore, these variations are extremely sensitive to the chemical compositions of the quasicrystals – with a spike in resistivity emerging at widely different temperatures, depending on which elements are present in the alloy, and in which proportions.
In their early experiments, Dr. Stadnik and his colleagues explored how this behavior is linked to quasicrystal “density of states”, which describes the number of different states at a particular energy level that the electrons within a solid material can possibly occupy.
To do this, the physicists measured ultrahigh-resolution spectra of ultraviolet photons, after being absorbed and re-emitted by the quasicrystals. They studied a wide range of metal alloys in the experiments – each containing three of a variety of elements including aluminum, copper, osmium, iron, and manganese, and in varying proportions. Their results both verified and discredited certain theories predicting the role of quasicrystal density of states – ultimately shedding new light on the atomic mechanisms involved in their loss in metallic behavior.
Probing Crystal Structures and Magnetism
Leading on from this work, Dr. Stadnik’s team next turned their attention to measuring the “magnetic susceptibility” of quasicrystals. Magnetic susceptibility describes the degree to which a material will become magnetized when exposed to an external magnetic field. To do this, they measured the diffraction of x-rays as they passed through the crystals – allowing them to precisely determine the geometric arrangements of their constituent molecules.
In addition, the researchers assessed the “Mössbauer spectra” of the materials, which measure the emission and absorption of gamma radiation by atomic nuclei bound within solids and are highly sensitive to even minute changes in the chemical environments of the nuclei. Through their experiments, Dr. Stadnik and his colleagues investigated metal alloys that were predicted to have particularly interesting magnetic properties, according to previous theories.
Again, the physicists investigated a wide variety of metal alloy quasicrystals in these studies. In one of the compounds they analyzed, the elements comprising the quasicrystal with five-fold rotational symmetry were replaced with compatible alternative elements – which the team expected would further alter its unique physical characteristics. The experiments were carried out at temperatures ranging from close to absolute zero, to room temperature.
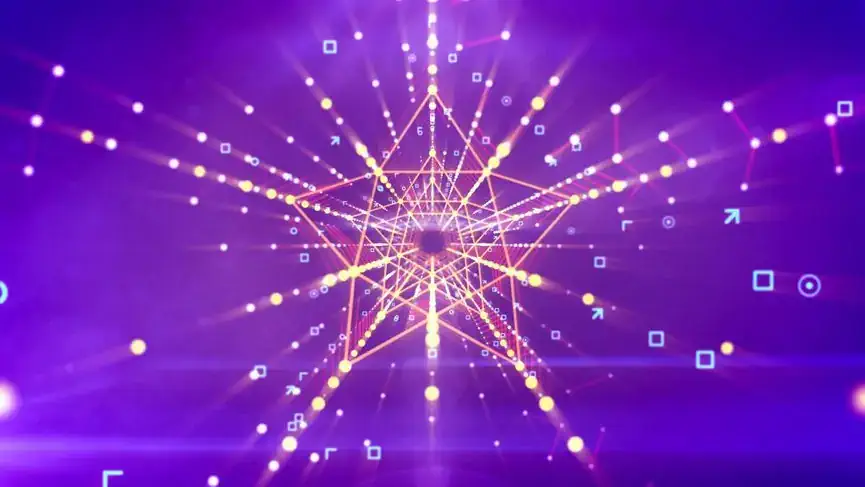
Frozen Out of Alignment
Above certain temperatures, Dr. Stadnik’s team determined that the quasicrystals behaved as ‘paramagnets’, which are weakly attracted to externally applied magnetic fields. Yet when they are cooled below these temperatures, the materials transform into ‘spin glasses’ – a term relating to the spins of quantum particles, including single atoms, which each point in a single direction.
When magnetized, the spins of all of a material’s constituent atoms will be aligned along a single direction. In spin glasses, however, these spins will become “frozen” while pointing in different, random directions, when cooled below a certain temperature. In the quasicrystals they analyzed, Dr. Stadnik and his colleagues observed that the directions of these spins fluctuated as the materials cooled, before freezing into a fixed final arrangement.
Altogether, these discoveries shed new light on the long-range magnetic fields generated by quasicrystals, and their fundamental differences with those produced by conventional crystals. Alongside studies of magnetization, Dr. Stadnik’s team also used Mössbauer spectra and x-ray diffraction to investigate the crystal structures of quasicrystals in more detail. From their results, they could identify how atoms of specific elements within quasicrystal metal alloys are clustered into dedicated sites, each with its own distinct geometries and atomic compositions.
Synthesizing New Structures
Dr. Stadnik’s discoveries so far have provided many important insights into the electrical and magnetic properties of quasicrystals, the molecular mechanisms that give rise to them, and the ways in which these characteristics can differ from those of regular crystals. But these experimental breakthroughs are only the beginning. Through their future research, Dr. Stadnik and his colleagues now aim to discover further novel properties by synthesizing many new families of quasicrystals.
The physicists will also carry out further investigation into the electrical and magnetic properties of quasicrystals, using a wide array of cutting-edge experimental techniques. Ultimately, they hope that this line of research will one day lead to a complete understanding of the fundamental properties of quasicrystals. Clearly, this will be of immense benefit to the wider field of condensed matter physics, and Dr. Stadnik hopes that it will enable experts in the field to find employment in leading industries and academic institutions.
Quasicrystals’ Applications in the Real World
Elsewhere, new advances in our understanding of quasicrystals, combined with the ability to engineer specifically desired physical properties, could have important implications for their use in industry, and even our everyday lives. Already, quasicrystals have been widely explored for their promising potential in a variety of real-world applications – including protective coatings that can better withstand external mechanical forces, and insulate against heat while barely taking up any space.
By embedding small particles of quasicrystals within specialized plastics, researchers are also hoping to design hard-wearing machine components such as gears, as well as durable prosthetics that can be safely integrated into the human body. Even further, quasicrystals are studied for their ability to selectively absorb certain wavelengths of solar radiation, allowing for the more efficient conversion from sunlight into electricity.
Dr. Stadnik and his colleagues now hope that through their focused efforts to engineer even more exotic structures and physical properties, these applications could soon be extended further still. With complete knowledge of the extent of their physical capabilities, their influence may one day expand into areas that physicists haven’t even yet considered.
Meet the researcher
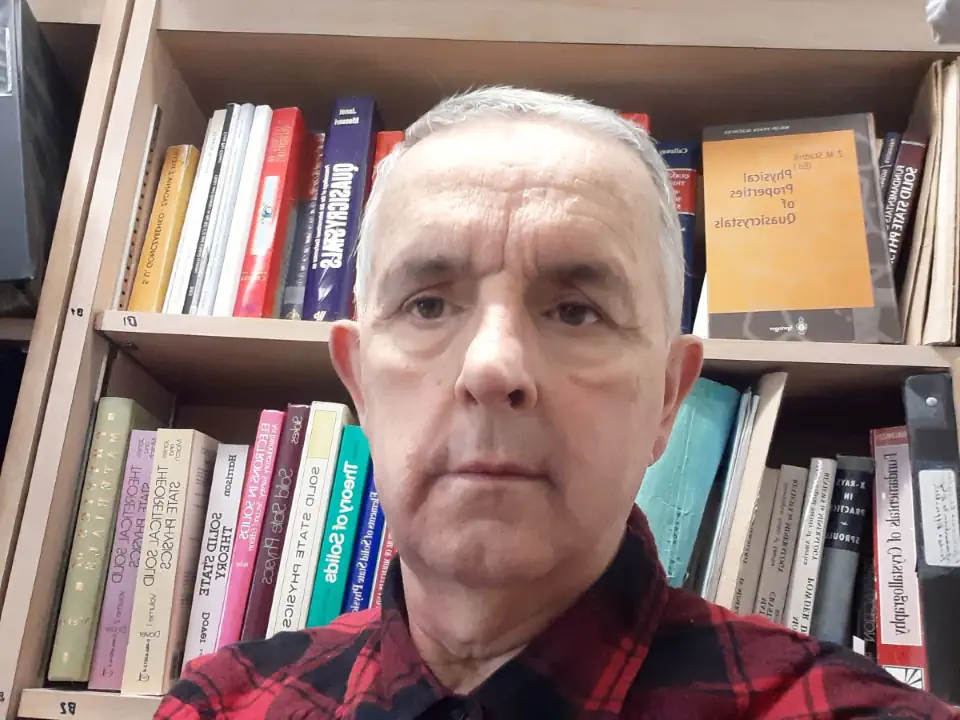
Dr. Zbigniew Stadnik completed his Ph.D. in Physics at Jagiellonian University, Poland, in 1980. He moved to Canada in 1986, where he first worked as a Research Associate in the Department of Physics at Dalhousie University. He joined the University of Ottawa’s Department of Physics in 1990, where he has been a full professor since 1997.
Dr. Stadnik’s research interests center around the discovery, design, and characterization of new metal alloys, including quasicrystals, metallic glasses, and geometrically frustrated magnets. He investigates these materials using a variety of cutting-edge experimental techniques, including photoemission spectroscopy, magnetometry, and Mössbauer spectroscopy.
Contact
Funding
NSERC (Natural Sciences and Engineering Research Council of Canada)
Further Reading
- F. Nejadsattari, Z. M. Stadnik, J. Przewoźnik, B. Grushko, Ab-initio, Mößbauer spectroscopy, and magnetic study of the approximant Al72Ni9Fe19 to a decagonal Al-Ni-Fe quasicrystal, Journal of Alloys and Compounds, 2016, 689, 726.
- P. Wang, Z. M. Stadnik, K. Al-Qadi, J. Przewoźnik, A comparative study of the magnetic properties of the 1/1 approximant Ag50In36Gd14 and the icosahedral quasicrystal Ag50In36Gd14, Journal of Physics: Condensed Matter, 2009, 21, 436007.
- K. Al-Qadi, P. Wang, Z. M. Stadnik, J Przewoźnik, Structural, magnetic, and Mössbauer spectral study of the icosahedral quasicrystal Zn77Fe7Sc16, Physical Review B, 2009, 79, 224202.
- Z. M. Stadnik, D. Purdie, Y. Baer, and T. A. Lograsso, Absence of fine structure in the photoemission spectrum of the icosahedral Al-Pd-Mn quasicrystal, Physical Review B, 2001, 64, 214202.
- Z. M. Stadnik, D. Purdie, M. Garnier, Y. Baer, A. P. Tsai, A. Inoue, K. Edagawa, S. Takeuchi, and K. H. J. Buschow, Electronic structure of quasicrystals studied by ultrahigh-energy-resolution photoemission spectroscopy, Physical Review B, 1997, 55, 10938.
- Z. M. Stadnik, D. Purdie, M. Garnier, Y. Baer, A. P. Tsai, A. Inoue, K. Edagawa, S. Takeuchi, Electronic structure of icosahedral alloys studied by ultrahigh energy resolution photoemission spectroscopy, Physical review letters, 1996, 77, 1777.
Source
This article originally appeared in Scientia, with the title of “Dr. Zbigniew Stadnik – Quasicrystals: Exploring an Exotic New Family of Materials”. Read the original article.
- An Innovative Medium-Voltage DC Circuit Breaker for Renewable Power Grids - October 13, 2022
- Scientists are Mapping the Earth’s Magnetosphere with Energetic Atoms - September 30, 2022
- Scientists are Developing Recyclable and Self-healing Plastics - August 9, 2022